Overview
Human papillomavirus (HPV) causes the prevalent sexually transmitted infection that accounts for the majority of cervical cancer incidences. Therefore, the development of a rapid, accurate, automatic and affordable nucleic acid detection strategy is urgently required for HPV tests, among which microfluidic chip is a promising diagnostic method.
In this work, we designed a microfluidic detection system consisting of a microfluidic chip and the corresponding detection using a Lateral Flow Assay strip to diagnose HPV 16. The proposed method integrates virus enrichment, DNA extraction, and real-time detection on a Lateral flow Assay strip into one device.
The integrated microfluidic detection system offers efficient and rapid diagnosis and demonstrates broad potential to deliver point-of-care detection in resource-limited circumstances owing to its simplicity and affordability.
MICROFLUDICIS TECHNOLOGY
Microfluidic technology enables a simple- and cost-saving route for molecular diagnostics during disease outbreaks compared with traditional laborious diagnostics. Compared with traditional diagnostics, microfluidic Point-of-Care Test utilizes miniaturized and portable devices, ranging from benchtop analyzers to small lateral flow strips, that conduct tests nearby the sampling sites. These tests are advanced for simplified or omitted sample preparation, rapid signal amplification, and sensitive signal readout, leading to a shorter testing duration producing accurate results. While developing these systems we must consider that they must be inexpensive, but also accurate, reliable, rugged and well suited to the medical and social contexts of the developing world.
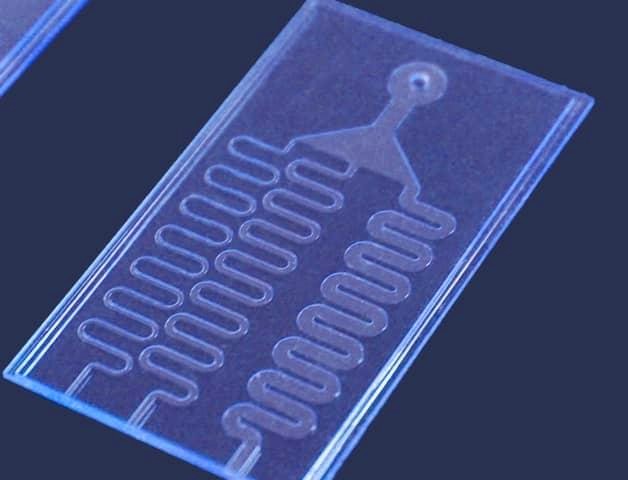
DEVICE ARCHITECTURE
A closed system based on microfluidics was designed which allows for a single-test setting, working with a small sample load and minimal chances of contamination. The basic build is made with a combination of PDMS layer and glass due to the biocompatibility of both materials. PDMS also known as Polydimethylsiloxane is mainly used in microfuidics devices as it is inexpensive compared to previously used materials (e.g. silicon). It is also easy to mold, as even when mixed with the cross-linking agent, it remains liquid at room temperature for many hours. It can mold structures at high resolutions[2]. The PDMS layer is combined with Borosilicate glass layer and is applied as a base plate for the microfluidic device due to its chemical resistance to liquids[3]. PDMS blockers are used to control the flow of the sample, buffer and reagents in and out of the microfluidics channel.
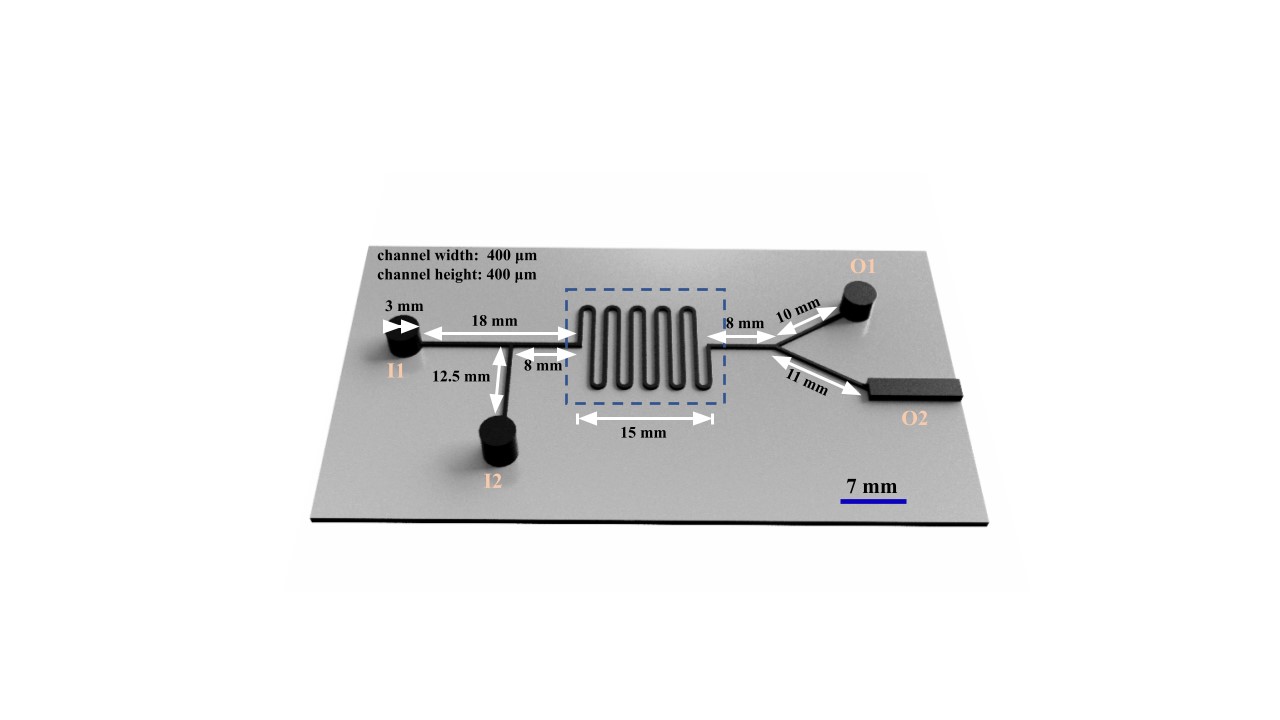
DIMENSIONS OF THE MICROFLUIDIC CHIP
The microfluidic chip was designed in AutoCAD fusion 360. The device consists of two inlets and two outlets with a serpentine flow channel in between
● Inlet Dimensions
The inlet I1 is designed to load the vaginal sample buffer while proteinas K and magnetic nanoparticles are loaded into the inlet I2.
The Channel I2 will be again used to load CRISPR reagents after washing the channel with a buffer. Channel width and height are fixed at 400 μm and the respective length for the sections are mentioned in the figure given below.
● Serpentine Channel
The serpentine channel with a total length of 115 mm is pre-coated with synbodies of peptides Annexin A2 and Alpha Integrin 6.
● Outlet Dimensions
The outlet O1 functions in the collection of residual volumes after each flushing step. The outlet chamber O2 has space for the loading of an LFA strip in the final step.
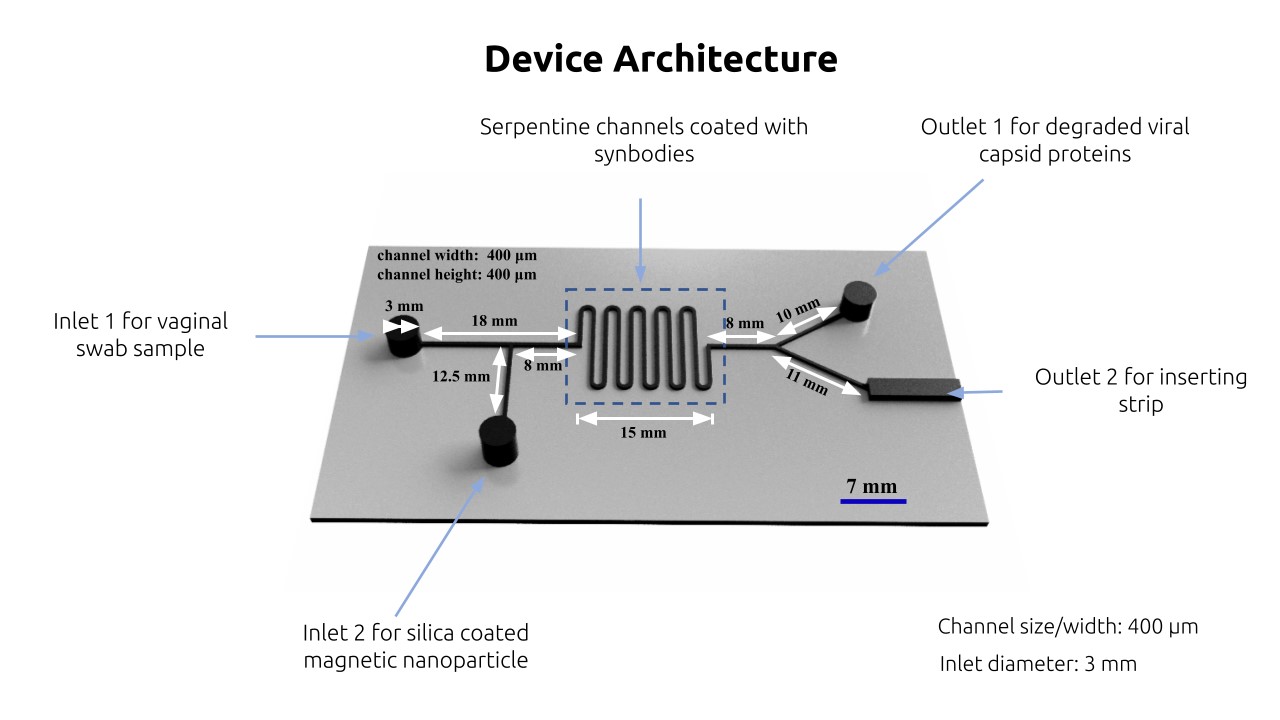
WORKFLOW
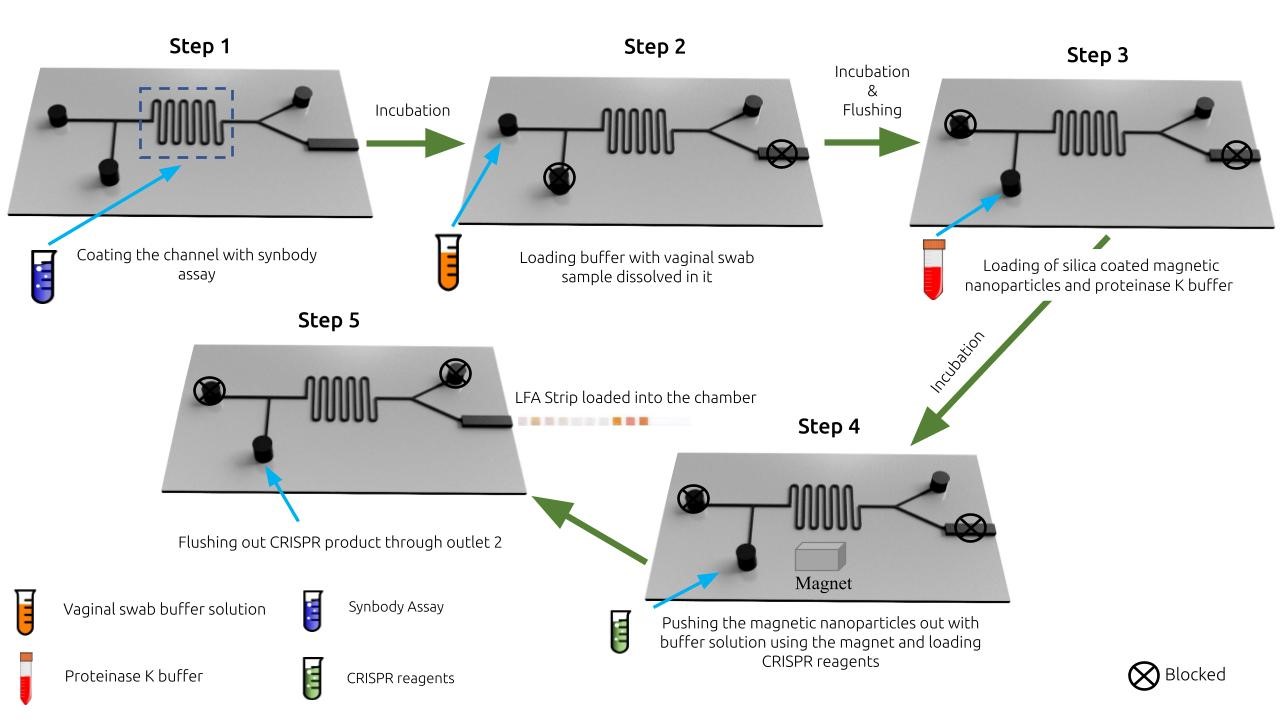
The whole process is divided into 5 steps with some steps being performed after a certain amount of incubation time :
1. The initial step is the loading of the vaginal sample through inlet I1 by using a micro-pipette. The amount to be injected is calculated such that the sample fluid fills till the end of the serpentine channel. This will ensure the interaction of the viral capsid in the sample with the pre-coated synbodies. During this initial loading, inlet I2 is blocked using a custom-made blocker patch.
2. After this, a solution of proteinase K and magnetic nanoparticles is injected from I2 which flushes the previously filled solution and also fills with the nanoparticles. During this operation, I1 is blocked.
3. Following incubation, a magnet is placed below the serpentine channel region of the microfluidic system after which the solution of degraded virus capsid is flushed using the pipette (I1 and O2 is blocked during this operation) which flows out through outlet O1. This step ensures the magnetic nanoparticles complexed with DNA stay within the serpentine channel region with the rest of the solution going out through O1.
4. The serpentine channel is then washed using a buffer to flush out the entire proteinase K solution and loaded with CRISPR reagents through I2 channel. With I1, I2 and O1 blocked the chip is incubated to complete the CRISPR reaction
5. In the last step, another buffer solution is injected through I2, carrying the CRISPR reaction products along and reaching outlet O2 where the Lateral Flow Assay strip is inserted and visual readout appears
HARDWARE PROTOCOL
The following reagents will be injected into the microfluidics chip using a pipette in the following order and quantities. Incubation time for each step will be similar to wetlab protocol for reaction at room temperature
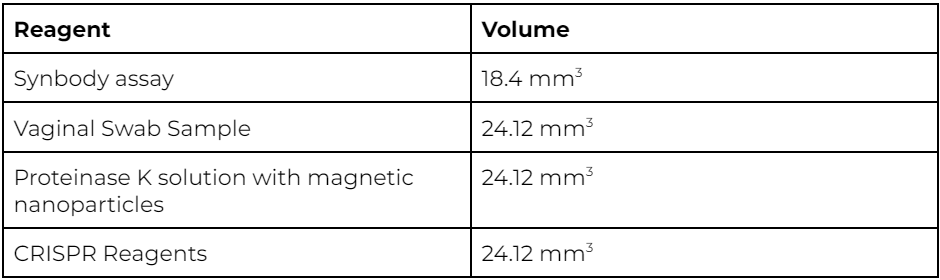
FUTURE DIRECTION
The industrialization of microfluidics is still in the start-up phase, reflected in complex channel design, expensive substrate materials, necessary optimization of reactions, liquid leakage, valve failure, and difficulty in reproduction and recyclability. These issues are the main barriers to large-scale adoption, so further improvement is needed to build a convenient chip-design platform, combined with material science to find less expensive substrate substitutes, enhance functional modularity, and push automation of chip production. Fortunately, in dealing with infectious diseases currently and in the future, microfluidic-based molecular diagnostic strategies are indispensable and receiving more attention from frontier scientists. Microfluidics-based molecular diagnostic strategies will become the mainstream of large-scale detection, utilizing rarely required samples, to conduct diagnosis automatically at a low cost
REFERENCES
1. Wang, X., Hong, XZ., Li, YW. et al. Microfluidics-based strategies for molecular diagnostics of infectious diseases. Military Med Res 9, 11 (2022). https://doi.org/10.1186/s40779-022-00374-3
2. Raj M, Kiran, and Suman Chakraborty. “PDMS Microfluidics: A Mini Review.” Journal of Applied Polymer Science, vol. 137, no. 27, Wiley, Jan. 2020, p. 48958. Crossref, https://doi.org/10.1002/app.48958.
3. Zhao X , Li X , Yang W , Peng J , Huang J , Mi S . An integrated microfluidic detection system for the automated and rapid diagnosis of high-risk human papillomavirus. Analyst. 2021 Aug 21;146(16):5102-5114. doi: 10.1039/d1an00623a. Epub 2021 Jul 15. PMID: 34264258.