Contributions
This year, our team encountered many challenges in executing our project. For instance, almost all of us are undergraduate students, who started their studies during the pandemic. So a lot of us have very limited experience in the lab. Another challenge was that we are very independent, in which we are not a part of any department of any of our universities. We lack advisors and hands-on PIs, making our project exceptionally challenging for us inexperienced undergraduate students. So for our contribution, we decided to create a summary of the very popular phylum of bacteria used in synthetic biology, cyanobacteria. Something that would have been exceptionally helpful kickstarting our project.
Most Common Cyanobacteria in Synthetic Biology
The most popular cyanobacteria in synthetic biology are Synechocystis sp., and Synechococcus elongatus.
Synechocystis sp.
Synechocystis sp. is another type of cyanobacteria that is commonly used as a model organism to study photosynthesis, carbon and nitrogen assimilation, evolution of plant plastids, and adaptability to environmental stresses. The strain PCC6803 is one of the most highly studied types of cyanobacteria as it can grow both autotrophically or heterotrophically in the absence of light. This specific strain is also known to have the ability to readily take up exogenous DNA. It is known to grow best in BG11 media, both liquid and solid medium. According to (Heidorn T. et al, 2011), its optimal growth conditions are at pH 7-8.5 , light intensity of 50 μmol photons m−2 s−1 and 1-2% carbon dioxide. Although carbon dioxide can optimize the bacteria’s growth, it is to be taken into account that there may be pH changes, therefore additional buffers would be recommended.
Heidorn T, Camsund D, Huang HH, Lindberg P, Oliveira P, Stensjö K, Lindblad P (2011). “Synthetic biology in cyanobacteria engineering and analyzing novel functions”. Methods in Enzymology. 497: 539–79. doi:10.1016/B978-0-12-385075-1.00024-X. ISBN 9780123850751. PMID 21601103.
Synechococcus elongatus
Synechococcus elongatus is a very similar cyanobacteria to Synechocystis sp., also commonly used in synthetic biology. At first, the most commonly used strain is the PCC 7942. But in 2016, a very similar yet better strain, UTEX 2973 was established, a unicellular cyanobacterium capable of rapid autotrophic growth (e.g. growth rate double than PCC 7942), comparable to heterotrophic industrial hosts such as yeast. It is especially great for transformation for facile generation of desired knockout and knock-in mutations. It is now known, that the optimal growth conditions would be 1.9 hrs in BG11 medium at 41°C under continuous 500 μmoles photons·m−2·s−1 white light with 3% carbon dioxide.
Yu, J., Liberton, M., Cliften, P. et al. Synechococcus elongatus UTEX 2973, a fast growing cyanobacterial chassis for biosynthesis using light and CO2. Sci Rep 5, 8132 (2015). https://doi.org/10.1038/srep08132
Engineering cyanobacteria
How to get foreign DNA into cyanobacteria?
Transformation
Transformation is the process in which bacteria incorporate free DNA from their surrounding medium. Bacteria that are capable of transformation are called naturally competent. Transformation has several biological functions but can also be employed for biotechnological purposes as naturally competent bacteria will simply uptake plasmids that they are incubated with. That means it is very simple and fast to introduce new features such as antibiotic resistances and enzymes needed for recombination or knock-out strategies.
An example for naturally competent cyanobacteria is Synechococcus elongatus PCC 7942. Recently, a strain of Synechococcus elongatus UTEX 2973, the fastest-growing cyanobacteria, has been modified in such a way that it gained the feature of natural competence from the first named species. [4]
Conjugation
Conjugation is the process in which bacteria directly exchange genetic material by forming bridge-like structures between two cells. It is the underlying mechanism of horizontal gene transfer and can also be biotechnologically used to introduce specific plasmids into cyanobacteria. Therefore, you need a triparental mating system: A donor strain containing a mobilizable plasmid, a recipient strain (here, the cyanobacteria) and a helper strain containing a helper plasmid for the conjugal DNA transfer. This method is especially useful if the cyanobacterial strain of interest is not naturally competent. [5]
Electroporation
Linear fragments of DNA can be transferred into cyanobacteria by electroporation. Cyanobacteria can be made electrocompetent by removing salt from the cell suspensions and by keeping them cold (< 4°C). [6]
Which options are there for modifying the genome of cyanobacteria?
λ Red Recombineering
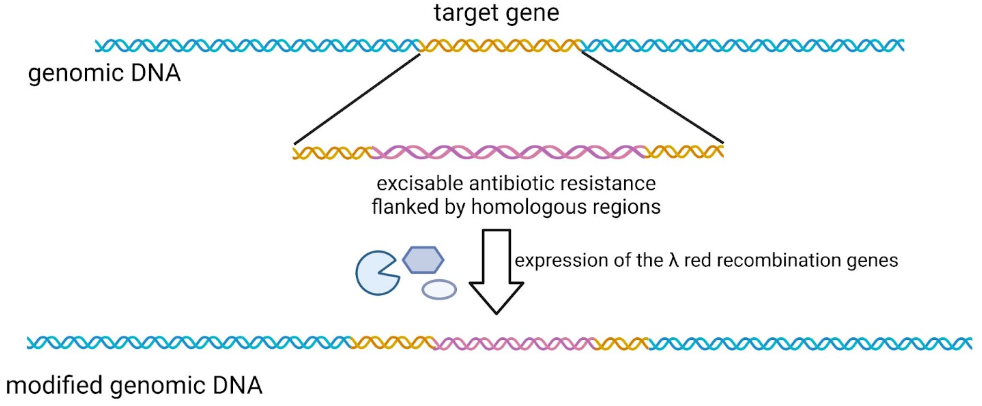
By the use of this strategy, one can realize knock-ins as well as knock-outs. For example, a certain gene can be knocked-out by replacing/disrupting it with an antibiotic resistance gene. If you use an excisable resistance cassette, you will be able to remove the resistance gene and get a clean markerless knock-out in the end. [8]
CRISPR/Cpf1 genome editing
This system can be employed to create knock-outs, knoch-ins or point-mutations. The first step is to construct editing plasmids. Backbone vectors containing the nuclease Cpf1 gene are available, the main task is to clone the framing segments of the target gene as guide RNAs into the vector. After that, the plasmid is transferred into the cyanobacterial target strain and its genome will be edited. In order to have a marker for a successful knock-out (apart from control via PCR), one could induce an antibiotic resistance or fluorescent protein by inserting the respective gene right after the guide RNA into the editing plasmid. [5]
Useful plasmids
Plasmids carrying antibiotic resistance genes
pKD3: carries ampicillin resistance and chloramphenicol resistance cassette flanked by FRT sites for excision with FLP recombinase [1]
pKD4: same as pKD3 but with kanamycin resistance instead of chloramphenicol resistance [1]
Plasmids for conjugation via the triparental mating system
pRL443: helper plasmid for mobilizing plasmids to cyanobacterial strains [7]
pRL623: helper plasmid for bacterial conjugal DNA transfer [7]
Plasmids for the λ red recombination system
pKD46: carries genes for λ red recombineering (inducible by arabinose) and ampicillin resistance gene, temperature sensitive (grow at ≤ 32°C to prevent plasmid loss, incubate at 37-42°C to ensure plasmid loss) [1]
pCP20: carries FLP recombinase gene (temperature inducible) and ampicillin resistance [2]
pSIJ8: backbone of pKD46, additionally carries FLP recombinase gene (rhamnose inducible)
→ combines features of pKD46 and pCP20
→ allows for a simplified and faster recombination protocol as only one plasmid has to be transformed into the target strain for all recombination events [3]
Plasmid for CRISPR/Cpf1 editing
pSL2680: base vector for constructing CRISPR/Cpf1 editing plasmids, carries kanamycin resistance gene [5]
Useful E. coli strains
In the last decades in the 20th century, different types of E.coli have been tested for their characteristics in the production of recombinant proteins. One derivative of the B line, BL21(DE3), has become the preferred host for recombinant protein production. This strain is thought to be fast growing because of the deletion of the fli gene, responsible for the biosynthesis of flagella. Furthermore, it is a preferred host for recombinant protein production due its deficiency of the key proteolytic system [9]. According to [10], the highest yield of pure protein could be achieved at a combination of factors (temperature 36.6 °C, time of harvest 7.5 h, speed of shaker 150 RPM and starting OD 0.1).
However, this strain is not suitable for maintenance of expression plasmids. For this, DH5α competent cells that do not carry the gene for T7 RNA polymerase (which BL21(DE3) carries) for plasmid DNA propagation would be recommended. Also XL1-Blue and HB101 competent E. coli are suitable for cloning strategies.
References
[1] One-step inactivation of chromosomal genes in Escherichia coli K-12 using PCR products. Datsenko KA, Wanner BL. Proc Natl Acad Sci U S A. 2000 Jun 6;97(12):6640-5. 10.1073/pnas.120163297 PubMed 10829079
[2] Cherepanov P. P., Wackernagel W. Gene disruption in Escherichia coli: TcR and KmR cassettes with the option of Flp-catalyzed excision of the antibiotic-resistance determinant // Gene. 1995. V. 158. No. 1. P. 9-14. PMID: 7789817
[3] Seven gene deletions in seven days: Fast generation of Escherichia coli strains tolerant to acetate and osmotic stress. Jensen SI, Lennen RM, Herrgard MJ, Nielsen AT. Sci Rep. 2015 Dec 8;5:17874. doi: 10.1038/srep17874. 10.1038/srep17874 PubMed 26643270
[4] Wendt KE, Walker P, Sengupta A, Ungerer J, Pakrasi HB. Engineering Natural Competence into the Fast-Growing Cyanobacterium Synechococcus elongatus Strain UTEX 2973. Appl Environ Microbiol. 2022 Jan 11;88(1):e0188221. doi: 10.1128/AEM.01882-21. Epub 2021 Oct 27. PMID: 34705549; PMCID: PMC8752150.
[5] Ungerer, J., Pakrasi, H. Cpf1 Is A Versatile Tool for CRISPR Genome Editing Across Diverse Species of Cyanobacteria. Sci Rep 6, 39681 (2016). https://doi.org/10.1038/srep3968
[6] Kallas, T. (2000). Cyanobacteria: Electrotransformation and Electroextraction. In: Eynard, N., Teissié, J. (eds) Electrotransformation of Bacteria. Springer Lab Manuals. Springer, Berlin, Heidelberg. https://doi.org/10.1007/978-3-662-04305-9_32
[7] Reduction of conjugal transfer efficiency by three restriction activities of Anabaena sp. strain PCC 7120. Elhai J, Vepritskiy A, Muro-Pastor AM, Flores E, Wolk CP. J Bacteriol. 1997 Mar;179(6):1998-2005. PubMed 9068647
[8] Murphy KC. λ Recombination and Recombineering. EcoSal Plus. 2016 May;7(1). doi: 10.1128/ecosalplus.ESP-0011-2015. PMID: 27223821.
[9] Rosano, G. L., Morales, E. S., & Ceccarelli, E. A. (2019). New tools for recombinant protein production in Escherichia coli: A 5-year update. Protein science : a publication of the Protein Society, 28(8), 1412–1422. https://doi.org/10.1002/pro.3668
[10] Tesařová, M., & Hrady, N. (2011). Optimization of growth condition Escherichia coli culture by method Design of experiments to produce optimal.
Example for a knock-out strategy in S. elongatus UTEX 2973-T
Abbreviated and modified from the following sources, here you can find step-by-step protocols:
Recombineering/Lambda red-mediated gene replacement – OpenWetWare
Protocol for gene knockout – Caroline Ajo-Franklin Research Group (lbl.gov)
Barrick Lab :: ProcedureFLPFRTRecombination
Jensen, S., Lennen, R., Herrgård, M. et al. Seven gene deletions in seven days: Fast generation of Escherichia coli strains tolerant to acetate and osmotic stress. Sci Rep 5, 17874 (2016). https://doi.org/10.1038/srep17874
Preparation of plasmids:
Grow up plasmids (pKD3 for Chloramphenicol resistance and pSIJ8 for λ red recombineering system) in host strains.
→ pSIJ8 is temperature sensitive, grow them at ≤ 32°C!
Perform minipreps to extract plasmids.
Preparation of target strain (containing the gene(s) to knock out):
Start overnight culture of target strain.
→ target strain needs to be naturally competent, e.g. S. elongatus UTEX 2973-T!
Take a sample of overnight strain; grow with fresh medium to a certain standardized culture density.
Wash with a transformation buffer, then add the transformation buffer.
→ Ice cold buffer to prevent further growth!
Preparation of linear DNA fragments with excisable antibiotic resistance gene:
PCR-amplify linear fragment from pKD3 (ChlR + FRT sites), check products on a gel (should be 1.1kb).
Transformation of the helper plasmid pSIJ8 into target strain:
Incubate competent cells on ice, add mini-prepped plasmid, heat shock and incubate on ice.
Spread on a plate with Ampicillin (to select successful transformants), grow overnight.
Making the target strain (now maintaining pSIJ8) electrocompetent:
Pick colonies and grow amp stock, add L-arabinose to induce pSIJ8 λ-red expression, and continue to grow.
Wash with ice cold dH2O in order to remove salt from the cell suspensions to prevent arcing
→ Cells must remain cold!
Electroporate linear PCR product into electrocompetent cells
→ target gene will be replaced with linear fragment (ChlR + FRT sites)
→ knock out of the target gene
Verify deletion of target gene/presence of antibiotic resistance and FRT sites with PCR
Remove antibiotic resistance
Induce pSIJ8 FLP recombinase expression by rhamnose.
Dilute, plate on LB, grow overnight.
Remove the helper plasmid pSIJ8 after final knock out
Incubate at 37-42 °C.
Screen for genomic recombination and plasmid loss
a) Test the colonies for Ampicillin and Chloramphenicol sensitivity.
→ Chloramphenicol: strain should be sensitive if removal of antibiotic resistance by FLP recombinase was successful.
→ Ampicillin: strain should be resistant because of pSIJ8; final strain should be sensitive after loss of pSIJ8
b) Do a colony PCR for scar (removed antibiotic resistance).
Grow some successful recombinants